The role of temperature in Solid
Phase Peptide Synthesis (SPPS)
In this case
study, we evaluate the effect of
temperature on the speed of synthesis
and crude purity
Introduction
When performing solid phase peptide
synthesis there are multiple factors
that contribute to the quality of the
produced peptide, the speed of the
synthesis, and solvent consumption. A
few factors include the coupling
chemistry, the amount of time for
deprotection and coupling phases as well
as the temperature of the synthesis
environment. A common question
researchers ask when inquiring about
automated peptide synthesizers is: “What
can I expect my crude purity to be?” and
“How long will it take to make a
peptide?”
In this case study, we break down how
the two most common chemistries used for
SPPS at different temperatures and
deprotection and coupling durations
affect peptide crude purity.
Brief
Solid phase peptide synthesis requires
a series of steps for each amino acid to
build the peptide chain: Deprotection,
washing, coupling, washing, repeat. At
CSBio we synthesized two peptides with
both DIC and HBTU chemistry with
different temperatures and cycles times,
and evaluated the effect on crude
purity.
All peptides were synthesized on Rink
amide resin at a 0.2 mmol scale on a
CSBio II Peptide Synthesizer. The
temperature was set on the CSBio II
Peptide Synthesizer, maintaining the
temperature of both the reaction vessel
as well as the pre-solvent delivery
(both the wash solvent and deprotection
solution are preheated prior to delivery
to the reaction vessel) at the
temperature noted in the table.
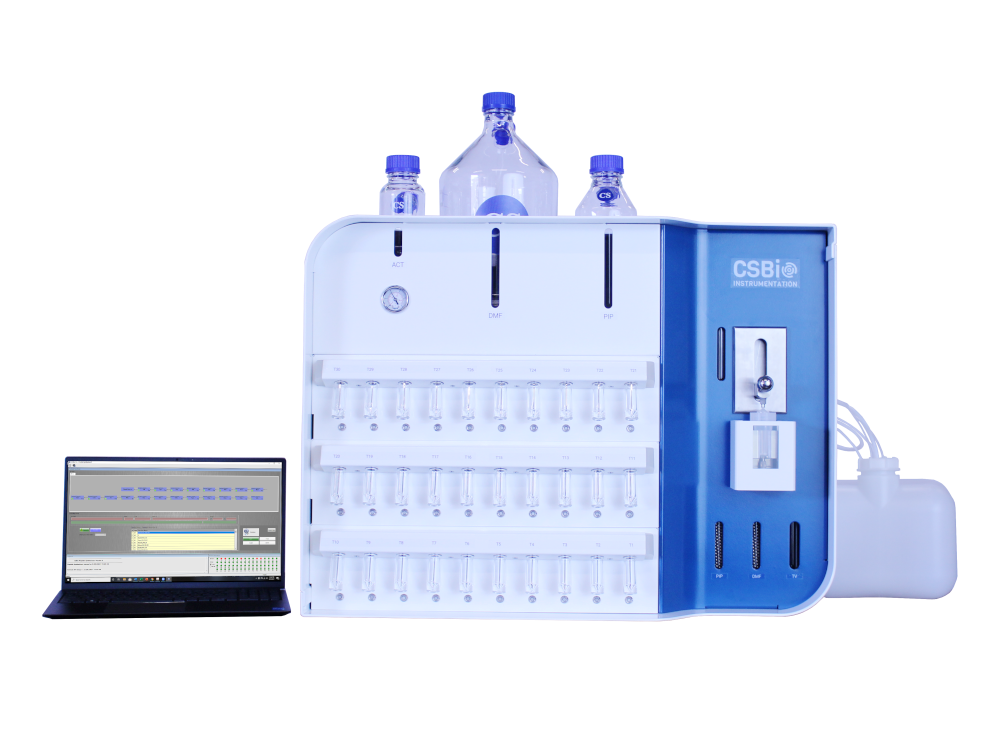
CSBio II Peptide Synthesizer
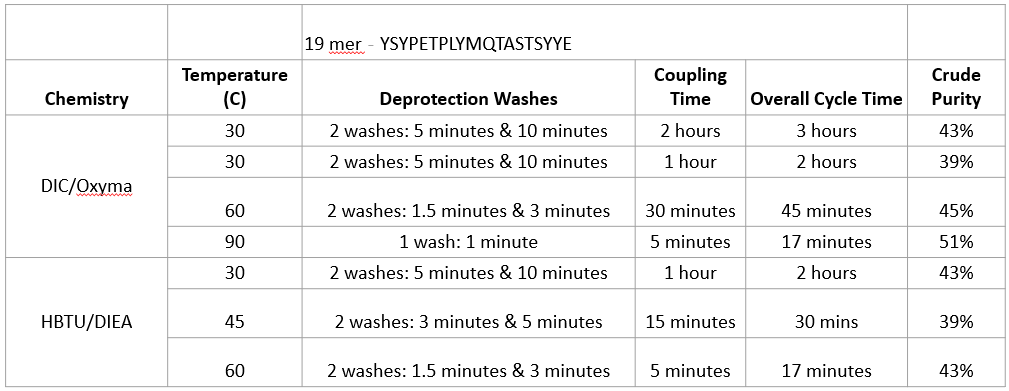
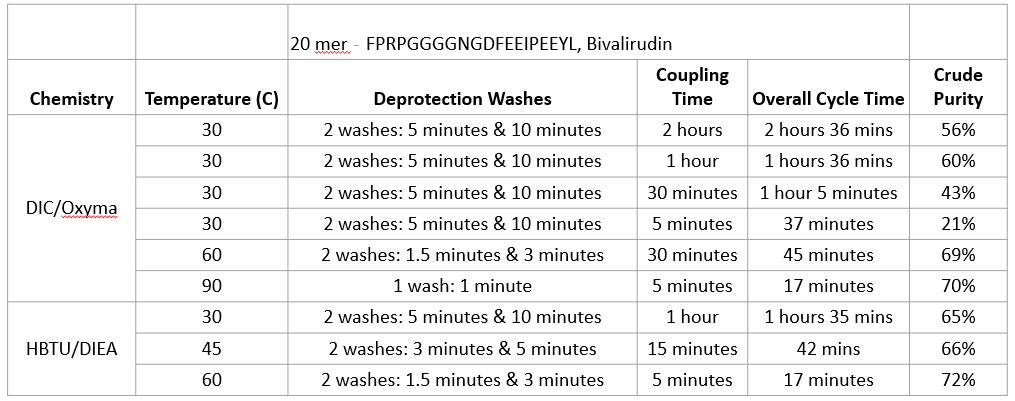
From the data, we can see that by
increasing the temperature, we are able
to reduce the coupling time to maintain
or even produce higher crude purity.
Additionally, if we maintain the same
temperature for the synthesis while
decreasing the coupling time, as
expected it will significantly
negatively impact the crude purity. As
evident more clearly as organized and
shown below with the crude purity
peaks.
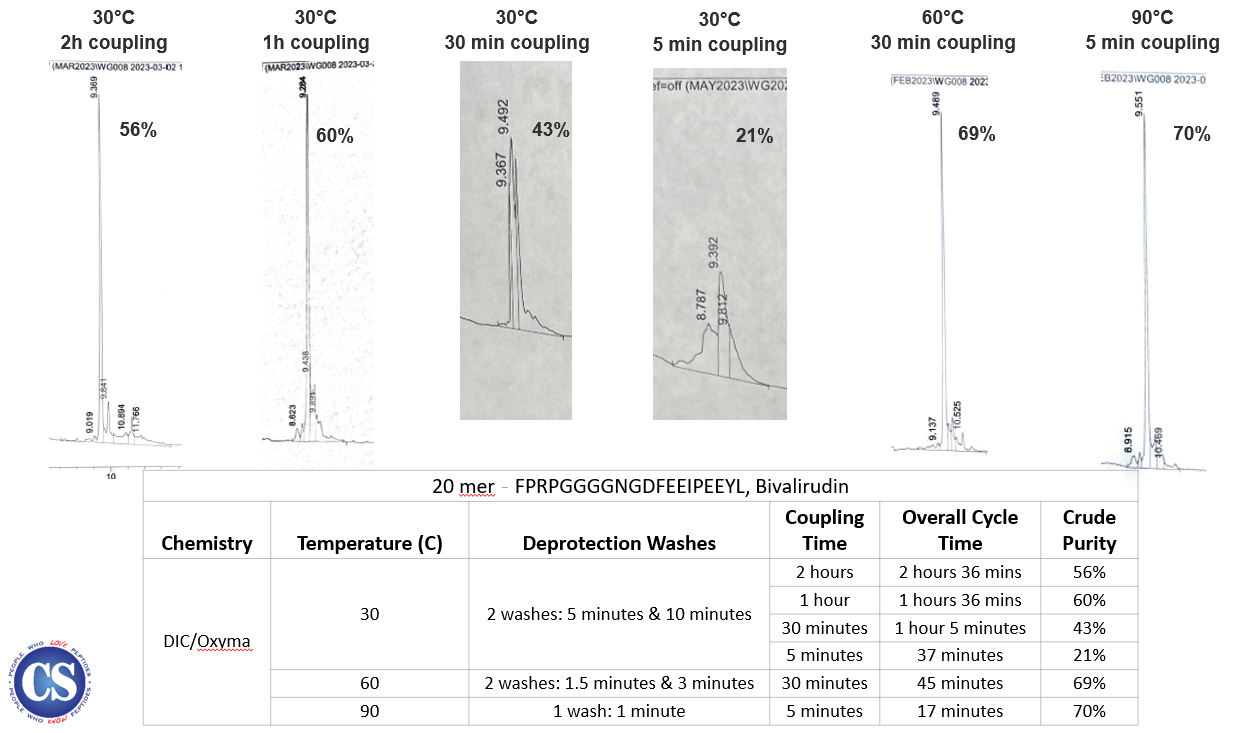
Increasing the synthesis temperature
is the best way to optimize the overall
cycle time, allowing users to shorten
the deprotection and coupling phases of
synthesis, regardless of the chemistry
or individual peptide sequence.
About CSBio For over 30 years, CSBio,
a leading peptide and peptide
synthesizer manufacturing company
located in Silicon Valley, California,
has been providing cGMP peptides and
automated peptide synthesizers to the
global pharmaceutical community. CSBio’s
peptide products and peptide
synthesizers can be found in production
laboratories, universities, and
pharmaceutical companies
worldwide.
Interested in any of our peptide
synthesizers? Get
in touch with
us.
Comparison of Microwave and
Conduction Heating for Solid Phase
Peptide Synthesis
In collaboration
with University of California, Davis;
UCD evaluated microwave and conduction
heating and presented the findings at
the 28th American Peptide
Symposium
Abstract
Solid phase
peptide synthesis (SPPS) has become a
standard approach for synthesis of
peptides, especially in a laboratory
setting. Heating the reactions in SPPS
could significantly reduce the coupling
and deprotection times. One of the
heating methods is to use microwave
which is becoming increasingly popular
because it not only dramatically reduces
the synthesis times, but also increases
the crude peptide purity [1]. However,
microwave peptide synthesizers are
relatively expensive. In this study, we
investigated whether SPPS using
conduction heating can achieve similar
result as microwave irradiation. CSBIO
II and CEM Liberty Blue were used as
heating resource of conduction and
microwave heating, respectively. Four
peptides with length of 18mer, 19mer,
20mer (Bivalirudin) and 39mer
(Exenatide) were selected as examples.
The peptides were synthesized using the
same synthesis protocol at 90 ºC
including identical coupling,
deprotection and washing cycles. The
differences between the two approaches
are the temperature of washing DMF (90
ºC vs 23 ºC for conduction and microwave
heating, respectively) and overall
synthesis cycle time (17 min vs 13 min
in conduction and microwave heating,
respectively). Both conduction and
microwave heating generated comparable
results with crude purity of 52.0% vs
51.7%, 49.0% vs 57.3%, 62.8% vs 57.1%,
37.0% vs 30.5% for 18mer, 19mer, 20mer
and 39mer, respectively. One of the
advantages of conduction heating is the
uniformly and consistently delivered
temperature during the synthesis which
could minimize racemization and side
reactions caused by spikes and hotspots
typically associated with microwave
heating. In addition, conduction heating
is also a more cost-efficient heating
method when compared to expensive
microwave heating technology.
Download the
full poster here Download
Link
About CSBio:
For over 30 years, CSBio,
a leading peptide and peptide
synthesizer manufacturing company
located in Silicon Valley, California,
has been providing cGMP peptides and
automated peptide synthesizers to the
global pharmaceutical community. CSBio’s
peptide products and peptide
synthesizers can be found in production
laboratories, universities, and
pharmaceutical companies
worldwide.
Interested
in any of our peptide synthesizers? Get
in touch with
us.
Synthesizing a 132-mer peptide
with
high purity
Using a CSBio
research scale peptide synthesizer, we
share a case study of how a 132-mer
peptide can be synthesized with high
purity
Introduction
Synthesis of
peptides at any length requires extreme
attention to detail for accuracy and
purity of the desired compound.
Correctly synthesizing peptides of 50
mer length is difficult, over 100 mer
length is a remarkable feat. CSBio’s
fully automated instruments are
purposefully designed to maximize resin
and solvent interaction while
maintaining a clean system throughout
the synthesis protocol, making it
possible to consistently synthesize
peptides containing over 130 amino acids
at very high purities.
Brief
The chemists
in CSBio’s in-house cGMP peptide
manufacturing facility were tasked with
making a cyclic peptide 132 amino acids
in length containing a Cysteine to
Cysteine disulfide bond linking the 39th
and 79th amino acids.
132 AA peptide sequence
(protected)
Using
a research scale CSBio peptide synthesizer,
Fmoc protected amino acids were attached one
by one in a stepwise fashion. Engineering
decisions such as 180° inversion mixing as
well as dedicated lines to separate the
delivery of amino acids and piperidine
ensure more resin to solvent interaction
than nitrogen bubbling, vortex or
oscillation mixing, while also maximizing
coupling with no risk of chemical cross
contamination.
CSBio CS136X
Upon peptide synthesis completion the
resin was cleaved giving the chemist’s
620mg of crude at 75% purity. A portion
of the crude (220mg) was loaded into a
1” C18 HPLC column, cyclization of the
fractions was performed using an iodine.
Post purification the chemists were able
to extract 18mg of the re-purified
disulfide peptide which tested at 98.7%
purity. Resulting in an 8.2% yield from
crude for a compound whose molecular
weight was >15000g/mol.
132mer post-purification
chromatogram
Conclusion
CSBio
synthesizers are designed to deliver the
user with the highest crude quality for
any possible peptide. As the only
automated synthesizer manufacturer that
produces cGMP peptides, constant
collaboration of in-house chemists and
engineers during the design process
enables CSBio to improve synthesis
results compared to industry
competitors; making CSBio synthesizers
the most robust and reliable instruments
on the market for synthesis of any
peptide.
Interested
in our research scale peptide
synthesizers? Get
in touch with
us.
A Practical Guide to Solid Phase
Peptide Synthesis (SPPS)
A 65 page guide
on the practical aspects of performing
SPPS
What
is this guide?
Written
through more than 60 years of combined
experienced in making peptides, this is
a 65 page practical guide on
SPPS.
The
purpose of this guide is two-fold.
First, a brief introduction on the
development and most common applications
of solid-phase peptide synthesis will
enable the user to best apply the two
most widely-used synthetic strategies –
Boc/Benzyl and Fmoc/tButyl chemistries –
to his/her projects. Second, a detailed
description of peptide synthesis,
cleavage, and purification in the
experimental section is given with
‘helpful hints’ so that newcomers to
peptide science will have easy access
and avoid some of the obstacles which
often lead to expensive mistakes and/or
poor synthesis yields
Introduction
Solid phase
synthesis is a process by which chemical
transformations can be carried out on
solid support in order to prepare a wide
range of synthetic compounds. This idea
was first developed by Bruce Merrifield
to synthesize polypeptides and earned
him the Nobel Prize in 1984. Solid phase
chemistry offers many advantages over
conventional synthesis in terms of
efficiency as well as convenient work-up
and purification procedures. In solution
phase peptide synthesis, particularly in
longer sequences, the repetition of
coupling and deprotection cycles can
become very labor intensive and require
the isolation of all peptide
intermediates.
I. A Brief
Historical Perspective The chemistry of
peptide synthesis – first developed in
the early 1900’s by Emil Fischer –
arguably marks the birth of organic
synthesis as we know it today. Whereas
the lion share of organic synthesis
continues to be performed by
solution-phase methods, i.e., with each
independent chemical reaction followed
by a purification step and
characterization of the resulting
synthetic intermediate, two
peculiarities of peptide chemistry
spurred the development of a more
efficient synthetic strategy. In
contrast to most total synthesis
efforts, the synthesis of peptides – at
least until the final deprotection step
– is an iterative process, with
alpha-amino (alphaN) deprotection and
amide couplings performed in succession
until the desired full-length target
peptide is obtained. In addition, most
peptides of biological interest are
grossly insoluble in most organic
solvents irrespective of side-chain
protection tactics. The net result of
these two characteristic features of
peptides was that the first sixty-odd
years of peptide synthesis forged little
ground until the landmark work in the
late 1950’s of R.B. Merrifield at
Rockefeller University.
During the
course of his Ph.D. work, Merrifield (a
biochemist) proposed an entirely new
paradigm in organic synthesis. As is
often the case when an outsider looks
into an insular field and pursues a tack
that is anathema to the existing experts
in the field, Merrifield’s idea of
performing all synthetic manipulations
using the C-terminus of the target
peptide linked to an insoluble solid
support was met with much criticism.
However, it was not long before the
chemistry of solid-phase peptide
synthesis (SPPS) was honed to a point
where traditional solution-phase
methodologies were no match with regard
to speed and versatility. The original
“Merrifield” version of SPPS – more
accurately referred to as Boc/Benzyl
chemistry – was roughly finalized in the
late 1960s, and employs a graduated acid
lability system for manipulation of all
protecting groups (Scheme 1). In this
strategy, the alpha-amino
t-butoxycarbonyl (Boc) protection is
removed with TFA, while side-chain
protections and the peptide- resin
anchorage (the linker) require much
harsher acidic conditions for cleavage.
This final step is accomplished using
liquid HF, a much stronger acid than TFA
(acidity functions of -11 and 0.1,
respectively).
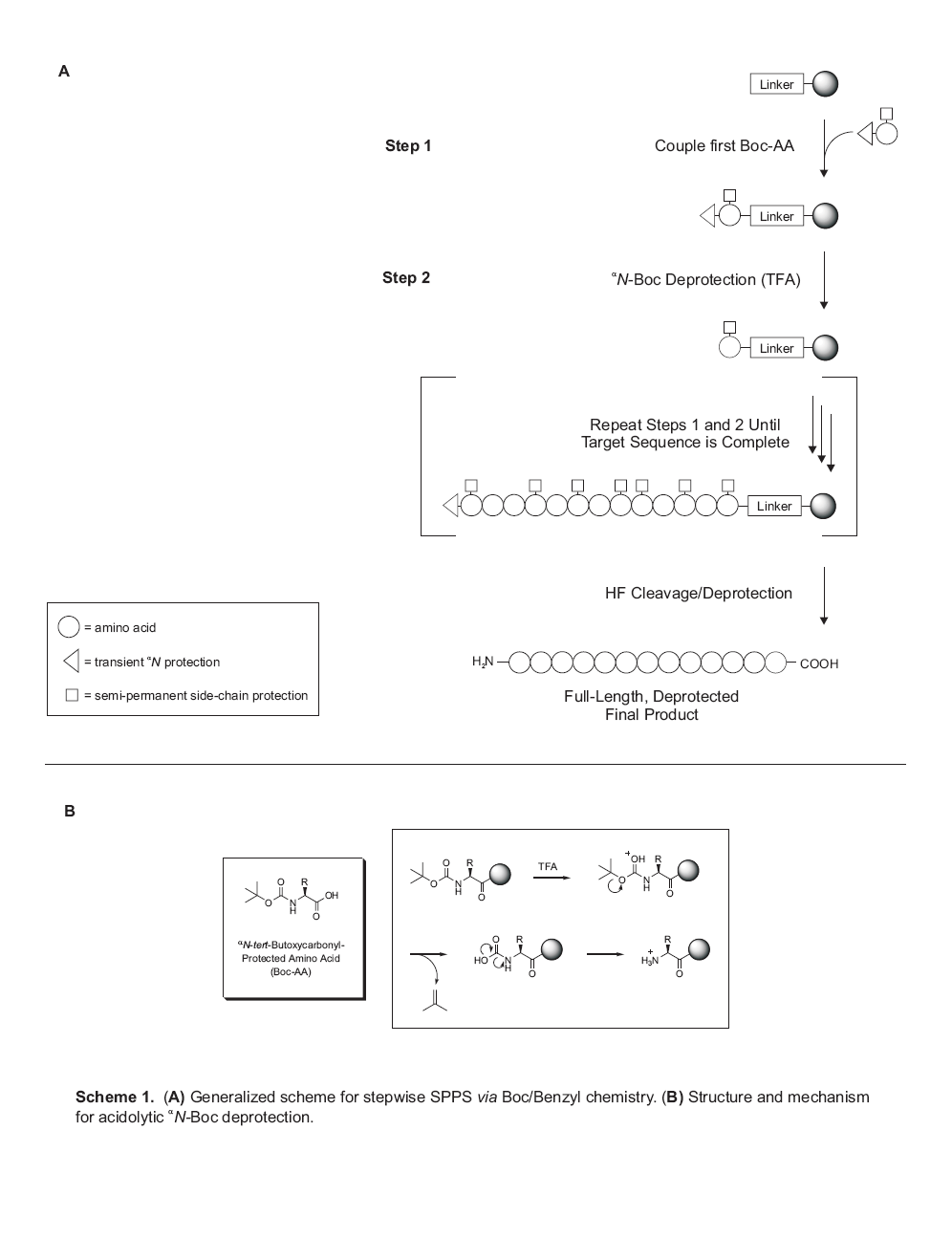
Scheme
1 depicts the manner in which Boc/Benzyl
SPPS simplifies all of the reactions
involved in peptide synthesis by
allowing for purification via
filtration, so that excess reagents can
be employed and removed by simple
washing. It is important to emphasize
that the chemistry of SPPS is not
fundamentally different from that used
in solution-phase peptide synthesis. The
sole chemical distinction between
solution- and solid-phase peptide
synthesis is that the C-terminal
protecting group in the latter is
rendered insoluble by virtue of its
incorporation into a polymer. This
difference notwithstanding, all
side-chain and alphaN protecting groups,
as well as coupling chemistries,
employed in solid-phase synthesis have
been successfully applied in
solution-phase synthesis, and
vice-versa, with few
exceptions.
During
the 1970s several groups were actively
developing milder methods for SPPS that
avoided the use of liquid HF as for the
final deprotection/cleavage reagent.
While a variety of milder graduated acid
lability systems were devised, the
method that rose to general
applicability was the orthogonal system
of Fmoc/tBu chemistry. This strategy –
developed by R.C. Sheppard at Cambridge
University – differs from Boc/Benzyl
chemistry in that the side-chain and
alphaN protecting groups are removed
under conditions that leave the other
class entirely intact. In Fmoc/tBu
chemistry, a mild base – usually
piperidine (pKa = 11.1) – is employed
for iterative N
9-fluorenylmethoxycarbonyl (Fmoc)
deprotection, while global side-chain
deprotection/cleavage is accomplished
with TFA (Scheme 2).
It
must be emphasized that the more
traditional Boc/Benzyl and Fmoc/tBu
chemistries, while differing in chemical
minutiae, are fundamentally the same
process. In both cases, the target
peptide chain is assembled in a stepwise
fashion from alphaN- and side-chain
protected amino acids. In both cases,
the ‘transient’ alphaN amino protection
is employed solely during chain
elongation (the coupling reaction) and
then removed for the subsequent coupling
reaction. Lastly, in both cases, the
final step – global side-chain
deprotection and cleavage of the
peptide-resin anchorage – is
accomplished by acidolysis, and the
target peptide isolated by trituration
from ether and purified by
reversed-phase high performance liquid
chromatography (RP-HPLC).
Download the
full 65 page guide here: Download
Link
About CSBio:
For over 30 years, CSBio,
a leading peptide and peptide
synthesizer manufacturing company
located in Silicon Valley, California,
has been providing cGMP peptides and
automated peptide synthesizers to the
global pharmaceutical community. CSBio’s
peptide products and peptide
synthesizers can be found in production
laboratories, universities, and
pharmaceutical companies
worldwide.
Research Scale Peptide
Synthesizers:
Demystifying Purity, Synthesis Speed
and Waste Generation
Understand what
drives peptide synthesis purity, speed,
and waste generation
Introduction
Anyone
that has performed peptide synthesis,
either manual or automated, realizes
quickly that each peptide is different.
Peptide sequences can vary in length,
contain many different amino acids or a
few of the same, be soluble or
insoluble, contain disulfide bonds, and
many more. With any standard synthesis
protocol, the “easy” peptide results in
both high crude purity and high purified
product yield. While a difficult peptide
using a standard synthesis protocol
delivers crude peptides with low purity
that require more downstream work and
lower yields of the purified product (or
in some situations, no mass
identification of the target
peptide).
When
performing peptide synthesis, it is not
uncommon for a peptide containing only
10 amino acids to be considered more
“difficult” to synthesize than a much
longer 25+ amino acid peptide. Overall
success with synthesizing a specific
peptide is generally impacted
by:
-
Peptide Length: the total number
of amino acids in the peptide
sequence.
-
Peptide Sequence: the specific
combination and order of the amino
acids in the sequence.
-
Synthesis Protocol: The set of
steps that together add amino acids
to the peptide sequence. These
include deprotection washes,
coupling time, as well as the number
of cleaning washes after both
deprotection and coupling.
Of
these factors, the Synthesis Protocol is
the only way one can control the
conditions of their syntheses and
optimize their protocol to deliver pure
peptides in a time-efficient
manner.
Peptide
Synthesis Protocols
When making
a peptide, users must balance the purity
and yield while also optimizing for
synthesis speed and reducing waste for
their workflow. Heating the synthesis
can allow users to lower the times for
deprotection and coupling, leading to
faster cycle times. This can be done in
a variety of ways including conduction
heating and microwave heating. For the
best results, heating needs to be
delivered uniformly and to consistent
temperatures. Conduction heating is
scalable from 20 mL up to
commercial-size reaction vessels 500L+.
It avoids racemization caused by spikes
and hotspots typically associated with
microwave heating. Conduction heating is
also a much more cost-efficient heating
method when compared to expensive
microwave heating technology.
Synthesis
speed and waste also go hand in hand as
shown in the chart. It is easy to see
that if a protocol contains more DMF
washes, 8 vs 3, the protocol with 8
washes will be expected to take longer
and generate more waste.
That bodes
the question: Why do two instruments
aiming to complete the same objective
differ so much in their standard
protocol?
Higher
Purity - The protocol with 8 washes is
more likely to deliver a higher purity
peptide due to maintaining a cleaner
synthesis. The washes after deprotection
are essential for ensuring that all the
Fmoc group is completely gone before
coupling. Doing washes after coupling
ensure that your main peak will be your
main peak, leaving no room for
cross-contamination between amino acid
cycles. Look at our case
study of the synthesis of a
132-mer peptide on CSBio’s
research scale peptide
synthesizers
Universally
Applicable - A user also benefits from
an automated peptide synthesizer that is
preloaded with protocols that will work
well on nearly all peptides, regardless
of sequence or length. The protocol with
8 washes can be run on a wide range of
peptide sequences and expect a
successful synthesis, not for a fraction
of the peptide sequences. Users of the
CSBio Peptide synthesizers can easily
choose between a conservative or
aggressive washing protocol giving them
the ability to reduce the number of
washings after deprotection and coupling
to improve synthesis speed and reduce
waste.
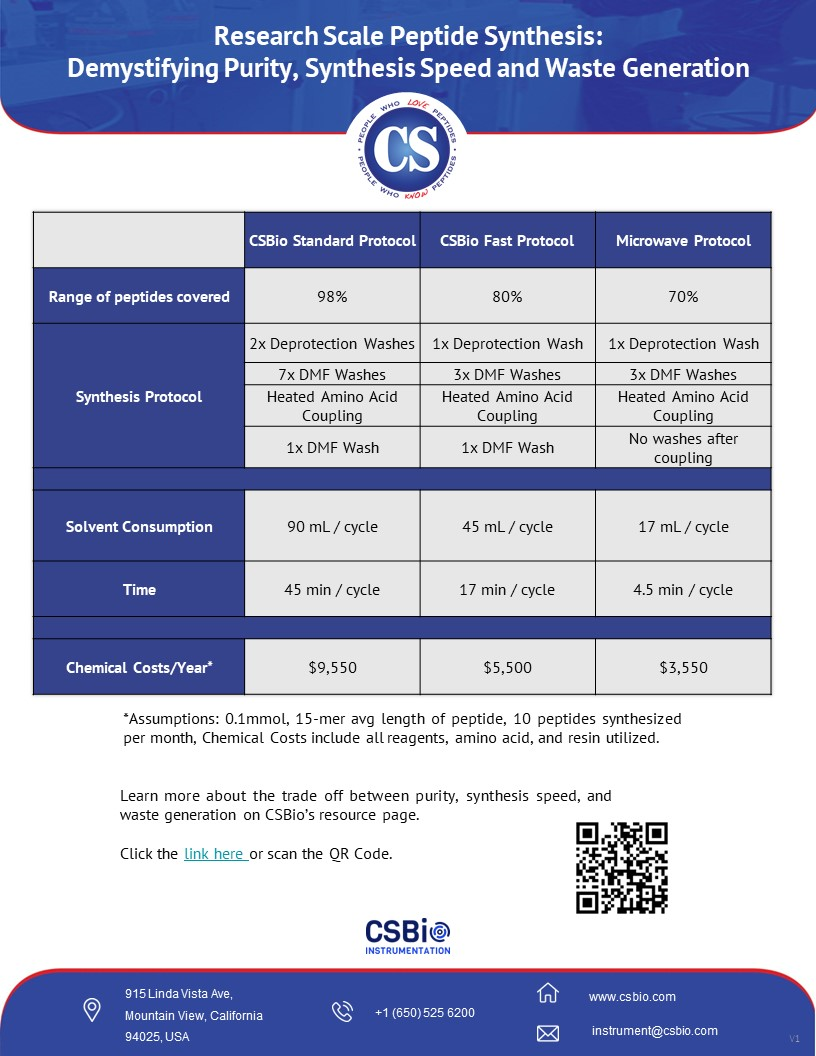
Peptide
Synthesis - Synthesis Protocols and the
impact on Purity, Speed, and Waste
Generation
Cost
to Perform Peptide Synthesis
What’s the
raw material cost to run a peptide
synthesizer? Well, most research labs
will synthesize ~10 peptides a month at
the 0.1 mmol scale, usually averaging
about 15 amino acids in length. Then
it’s largely dependent on the synthesis
protocol utilized and the type of raw
materials used. Some peptide synthesizer
manufacturers require special amino
acids and resins to run their synthesis
protocol, such as His(Boc) that can be 7
times more expensive than standard
His(Trt), or PEG-PS resin that can be
twice as expensive as Rink Amide MBHA
resin.
When
considering the synthesis protocols, the
amino acids, resin, and all the reagents
and solvents, a typical cost per year
will range between $5k to $10k. This
considers purchasing the amino acids in
100g bulk (not pre-packaged cartridges),
and does not include any disposable or
consumable items that the synthesizer
manufacturer may require. If you're
interested in learning about the details
of the cost to perform peptide synthesis
and these calculations, just reach out
to us.
As users can
see, while there may be a marginal
potential cost savings from the reduced
waste of the microwave protocol, this
doesn’t factor in re-running the
synthesis in situations that the target
peptide is not found. Even without the
re-synthesis situation, looking at the
total cost per run is negligible when
factoring in the much higher purchase
price of an expensive microwave
synthesizer.
With a CSBio
Peptide Synthesizer, users are confident
that the provided protocols deliver
high-quality results on difficult
peptides while allowing users to easily
change the protocol to manage synthesis
speed and waste. Reach out to us at instrument@csbio.com,
schedule a
call to learn more,
or just ring us directly at +1 650 525
6200 if you’re interested in discussing
your peptide synthesizer needs.
About CSBio:
For over 30 years, CSBio,
a leading peptide and peptide
synthesizer manufacturing company
located in Silicon Valley, California,
has been providing cGMP peptides and
automated peptide synthesizers to the
global pharmaceutical community. CSBio’s
peptide products and peptide
synthesizers can be found in production
laboratories, universities, and
pharmaceutical companies
worldwide.
From peptide drug discovery,
through
process development, to commerical
scale manufacturing
In this case
study, we talk about CSBio's peptide
synthesizer platform
and knowledge that allows companies to
utilize our peptide synthesizers during
the early days of drug discovery, all
way through commerical scale
manufacturing
Introduction
When
a multinational pharmaceutical company
initiated their in-house
manufacturing of peptides in the early
phases of drug discovery, they came to
CSBio for their instrumentation needs.
As a manufacturer of custom peptide
synthesizers from small scale to large
scale manufacturing, this pharmaceutical
company knew they had a partner that
could meet all of their instrumentation
needs from drug discovery to commercial
manufacturing for process development
and scale up ease.
Brief
During drug
discovery
for lead optimization, this
pharmaceutical company determined they
needed a
reliable small scale synthesizer to
produce peptide analogs which would be
used
in testing to determine the best analog
for scale-up and clinical trials. As a
fair amount of material would be
required for this testing (20-100 mg)
and
flexibility in the synthesis protocol
was important, they turned to the CS136
which enabled their discovery lab to
rapidly synthesize dozens of peptide
analogs in a short amount of time with
enough material produced for multiple
tests and assays.
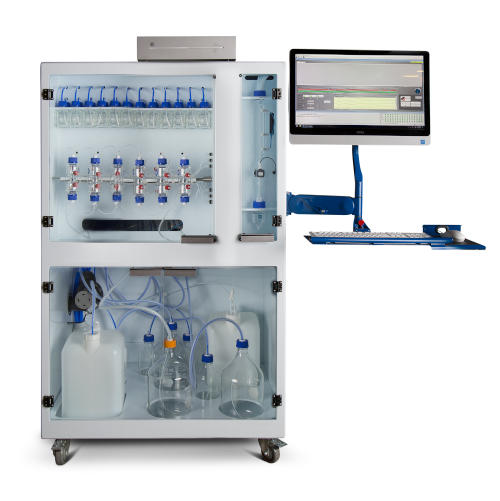
CSBio
CS136M
Once
a primary peptide drug candidate was
selected, the peptide chemists
at this pharmaceutical company were
tasked with making a continuous supply
of
high purity peptide for toxicological
studies, PK studies, formulation work
and
additional assay testing. Grams of
peptide were now required, so the
pharmaceutical company set up a CS536
system in the process development
laboratory to accomplish this
task.
To
manage the scale up transition from
small scale to large scale
manufacturing, a second CS536 system was
purchased shortly thereafter and
customized with both a 180° inversion
mixing system and overhead stirring.
This
unique design was a collaboration
between CSBio design engineers and the
pharmaceutical company to add an extra
layer of confidence in their methodology
as they prepared for the scale up
transition from inversion mixing to
overhead
mixing, which would ultimately be
required for large scale manufacturing
of the
peptide drug.
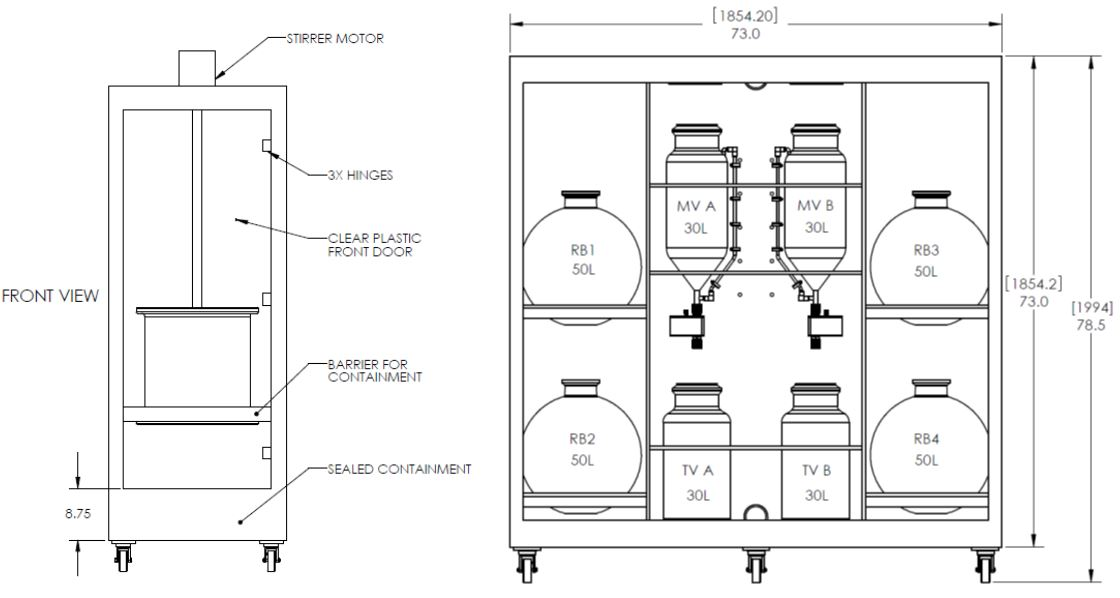
Custom
Designed CSBio CS936
As the
pharmaceutical
company moved into late stage clinical
trials the new task at hand was scaling
up for commercial manufacturing. CSBio
and the pharmaceutical company worked
closely together to design a CS936
production scale synthesizer that would
suit
their exact large scale production
requirements. It was imperative that the
transition from R&D scale to process
scale to manufacturing was as seamless
as possible. As all CSBio synthesis
systems use the same 21 CFR Part 11
compliant CSPEP software®, the
transition from milligrams to grams to
kilograms
was made much easier as the synthesis
protocols could be transferred up the
production scale.
The CS936
unit was synthesizing
manufacturing runs immediately upon
installation/operational qualification
as
there was virtually no learning curve
for the manufacturing group. CSBio has
purposely designed our systems to make
scale up from R&D to commercial
manufacturing as easy as
possible.
Conclusion
CSBio
provides customers with peptide
synthesizers that fit their exact
needs throughout the lifecycle, whether
it’s during early drug discovery or
late stage commercial manufacturing. By
working intimately with customers
coupled with over 30 years of peptide
and instrumentation knowledge, customers
can trust they have a partner to ensure
a successful project.
Interested
in any of our peptide synthesizers? Get
in touch with
us.
Research Scale Peptide Synthesizer
Comparison - A Guide to Choosing a
System that Fits Your Needs
We've gathered the key
things to know to adequately compare the
most popular synthesizers on the
market for users searching for a peptide
synthesizer.
Introduction
A major
reason medicinal
chemists and researchers love peptides
is they offer a unique ability to treat
unmet clinical needs in comparison to
small molecules and biological
therapeutics. With over 70 approved
peptide therapeutics on the market
treating
patients today, researchers are looking
for the next peptide therapeutic that
will make waves.
What about
synthesizing
the peptides that researchers use for
their studies? CSBio has been making
peptides and peptide synthesizers for
over 30 years, and we’ve developed
synthesizers for the first-time peptide
synthesis user as well as the advanced
peptide chemist. Here’s the major things
to consider when comparing research
scale peptide synthesizers for your
needs.
1. Manual
or Fully
Automated?
This day and
age, a
fully automated synthesizer is table
stakes. But making sure the synthesizer
you buy is fully automated is the most
important factor.
For those
that haven’t
made a peptide before, a typical solid
phase peptide synthesis protocol will
consist of deprotection cycles, multiple
washes, coupling, and more multiple
washes for each amino acid addition to
their peptide. Dependent on the peptide,
there can be multiple washes and
deprotection cycles to ensure adequate
synthesis, defined by high purity of the
targeted peptide being
synthesized.
Typical
Peptide
Synthesis Cycle for each Amino Acid
Addition:
2x
Deprotection
6x DMF
Wash
Coupling
2x DMF
Wash
When
performing manual
synthesis, the washes and deprotection
cycles are extremely redundant and time
consuming, requiring manual solvent
addition and draining for each wash or
deprotection cycle. Semi or manual
peptide synthesizers typically only
perform
the coupling step, and require users to
manually perform the remainder of the
synthesis. Given the coupling step is
the least labor intensive portion of the
synthesis and accounts for less than 10%
of the touch time, these peptide
synthesizers are categorized as manual
synthesizers.
What does it
mean to
have a fully automated peptide
synthesizer? Most peptide sequences are
around
20 amino acids long, and users would
like to be able to setup and walk away
from their peptide synthesizer and come
back to a completed peptide. This means
the synthesizer is able to fully
automate the completion of a synthesis
cycle
for each amino acid, as well as continue
to subsequent amino acids without any
user intervention such as adding more
solvents, reagents, or amino acids.
Having a fully automated synthesizer
that allows users to make an entire
peptide without any manual steps in
between is by far the most important
factor
to verify when buying a peptide
synthesizer.
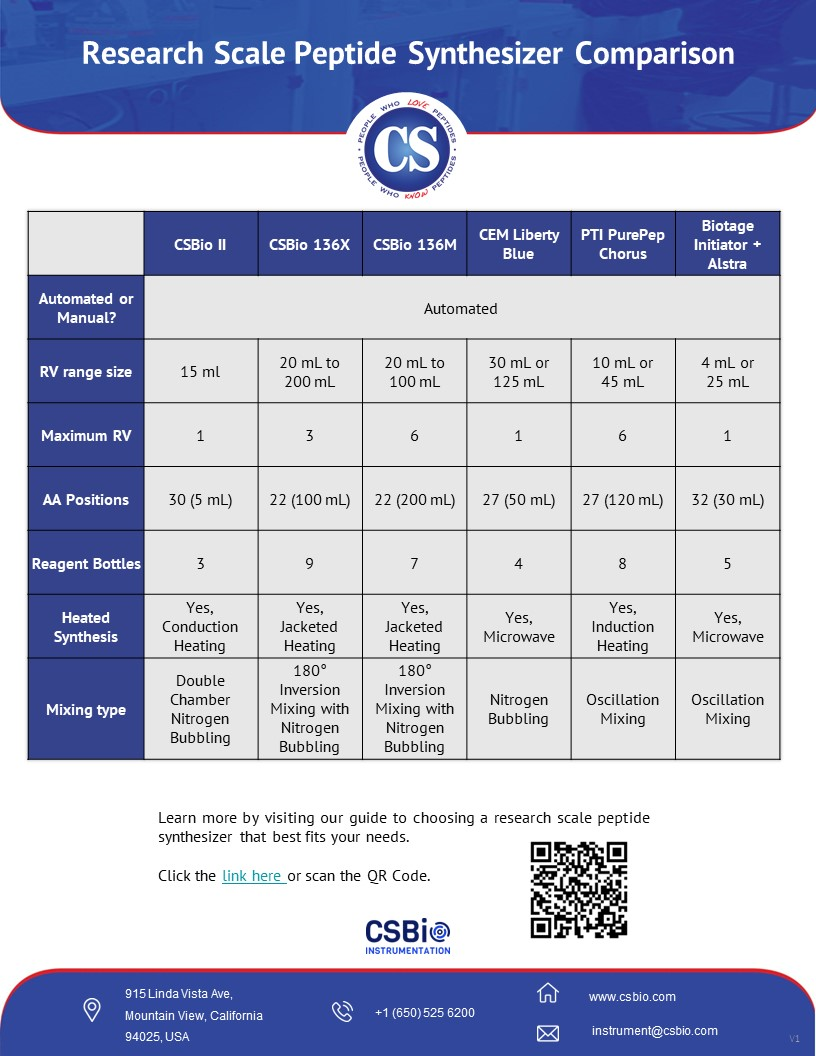
Comparison
of Popular Research Scale Peptide
Synthesizers
Take a look
at our case
study of the synthesis of a
132-mer peptide on CSBio’s
research scale peptide
synthesizers,
which was performed entirely unattended
without any solvent
recharging, amino acid additions, or
manual steps.
2.
Reaction Vessel (RV)
Size
The reaction
vessel size
determines the maximum synthesis scale
that a peptide synthesizer is capable of
performing, and synthesis scale
determines the amount of peptide
material that
can be produced per synthesis batch.
Most peptide synthesizers consider the
solvent, reagent, and amino acid
consumption based on the reaction vessel
size
to ensure adequate unattended
automation; as users would not want a
reaction
vessel sized on a synthesizer that
requires solvent addition every 30
minutes.
Dependent on
what
quantities (mg to grams) a user requires
at their phase of research, determines
the size of reaction vessel. The amount
of peptide that can be produced in any
given reaction vessel can depend on many
factors including resin substitution,
chemistry, number of amino acid
additions, and expected growth. Some
manufacturers only talk about synthesis
scale based on mmol, but it’s difficult
to compare when there are so many
factors that determine mmol outside of
reaction vessel size. To provide a rough
idea, a 15ml reaction vessel can be
used to produce anywhere from 50mg to
500mg dependent on these many factors.
For a 20-mer peptide, using 0.4
mmol/gram substitution resin, a 0.1mmol
synthesis can be performed with a 15ml
reaction vessel producing ~300mg of
crude peptide. Upon purification,
dependent on purity requirements, this
crude
peptide can generally yield ~100mg of
pure peptide.
3.
Purity, Synthesis
Speed, and Waste
Generation
After
determining the
quantity of peptide required, a few
other practical questions to ask when
deciding on a peptide synthesizer is how
many peptides will you make per month,
and at what purity will you need for
these peptides? Most research scale
peptide
labs are synthesizing around 10 peptides
per month, and purity requirements can
vary but 90 to 95% is typical. With
these requirements, ensuing a high
quality
crude peptide leads to ease of
purification.
What are the
factors
that drive a high quality crude peptide?
It comes down to the peptide amino
acid sequence, the length of the
peptide, and the synthesis protocol.
With an
“easy” peptide, a fast protocol with low
waste generation can yield a high
quality crude peptide, while a
“difficult” peptide with that same fast
protocol
can at times not even yield the correct
target peptide (as identified by mass
spectrometer) within the crude
product.
The
synthesis protocol
itself is also the primary driver when
it comes to synthesis speed and waste
generation. As such, many times there’s
a trade-off between synthesis speed,
waste generation, and purity. Take a
look at our article Demystifying
Purity, Synthesis Speed
and Waste Generation that talks in detail about this.
4.
Chemistry Flexibility
For most
users making
peptides, the desired goal is to simply
produce their target peptide, where
having an easy to use peptide
synthesizer is more desirable than
having a lot
of features which can increase
flexibility, but also add
complexity.
For the
advanced peptide
chemist, there may be a desire to have a
lot of flexibility in performing
different types of chemistry to improve
synthesis yield, or to be able to
develop a process for scale up. This
flexibility could be performing double
couplings, cappings, doing Fmoc
synthesis, Tboc synthesis, using DIC
coupling
or HBTU coupling within the same
peptide, to name a few.
While there
are many
detailed factors to consider that will
drive this flexibility, primarily
related to the software interface and
how system functions are performed, a
major factor is whether the system has
the dedicated reagent bottles available.
To perform DIC coupling on one amino
acid addition, followed by HBTU coupling
on the subsequent amino acid addition in
the peptide sequence, requires the
dedicated reagent bottles to be able to
perform this in a fully automated
manner.
These are
the top things
to consider when looking for a research
scale peptide synthesizer. There are
many other considerations as well, such
as:
Cost of the
synthesizer,
as well as cost of long term ownership
when it comes to reliability and ease of
repair and maintenance
Performing
chilled and
heated synthesis
Performing
high
throughput synthesis if a large quantity
of peptides is required on a weekly
basis.
Scale up
considerations,
where users will desire to establish
processes on research scale systems to
move towards pilot and commercial scale
peptide production.
Reach out to
us atinstrument@csbio.com,
schedule a
call to
learn more, or just
ring us directly at +1 650 525 6200 if
you’re
interested in discussing your peptide
synthesizer needs.
About CSBio:
For
over 30 years, CSBio,
a leading peptide
and peptide synthesizer manufacturing
company located in Silicon Valley,
California, has been providing cGMP
peptides and automated peptide
synthesizers
to the global pharmaceutical community.
CSBio’s peptide products and peptide
synthesizers can be found in production
laboratories, universities, and
pharmaceutical companies
worldwide.
|